Flow chemistry, also known as continuous flow or microreaction technology, involves conducting chemical reactions in a continuously flowing stream rather than in traditional batch reactors. This approach offers significant advantages for the synthesis of Active Pharmaceutical Ingredients (APIs), including enhanced control over reaction conditions, improved safety, and increased efficiency.
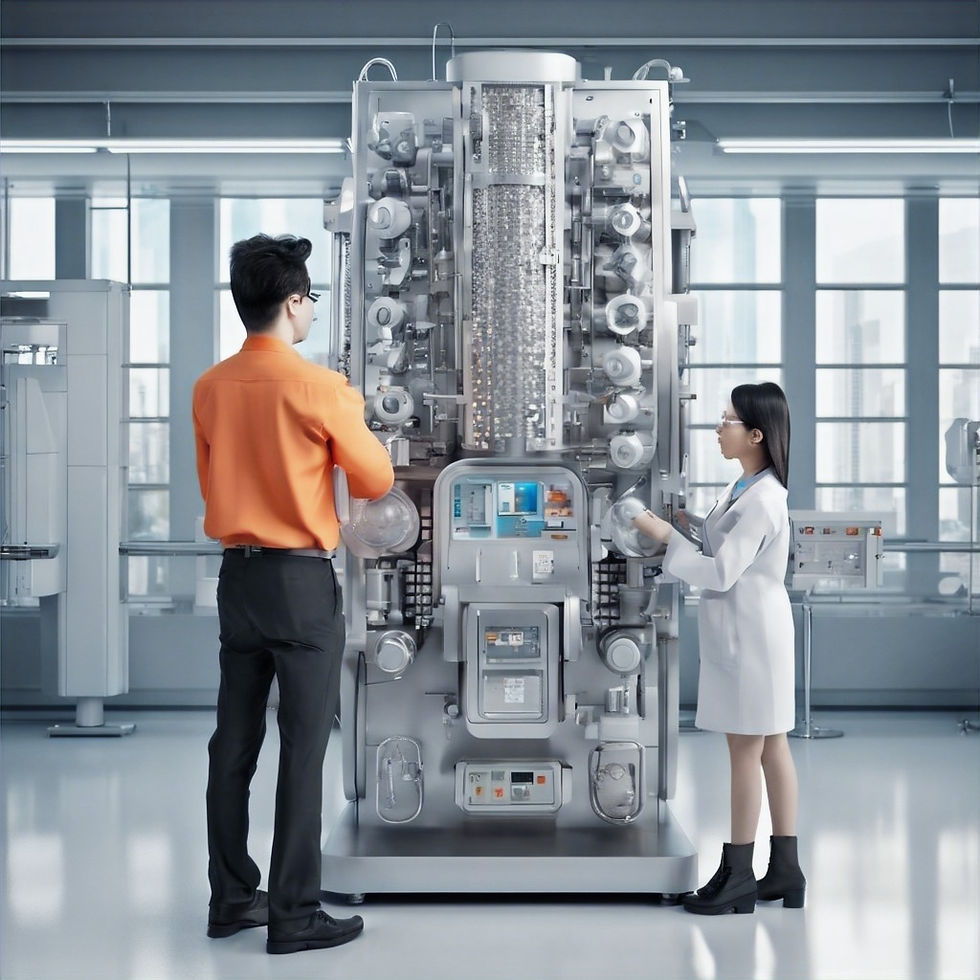
Here are the key aspects of using flow chemistry for API synthesis:
1. Advantages of Flow Chemistry
1. Enhanced Control and Precision
- Consistent Reaction Conditions: Flow reactors provide precise control over reaction parameters such as temperature, pressure, and reaction time, leading to consistent and reproducible outcomes.
- Improved Mixing and Heat Transfer: The small dimensions of flow reactors enhance mixing and heat transfer, which can improve reaction rates and yields.
2. Safety Improvements
- Handling Hazardous Reactions: Continuous flow systems allow for safer handling of hazardous reagents and reactions, as only small quantities of reactants are in the reactor at any given time.
- Controlled Environment: Flow chemistry minimizes the risk of thermal runaways and enables safe scaling of exothermic or potentially explosive reactions.
3. Increased Efficiency and Scalability
- Continuous Production: Flow chemistry enables continuous production of APIs, which can lead to higher productivity and reduced manufacturing costs.
- Scalability: Reactions developed in flow can be scaled up more easily than batch processes, often by running multiple flow reactors in parallel.
4. Sustainability and Green Chemistry
- Reduced Waste: Enhanced reaction efficiency and minimized solvent use contribute to greener processes.
- Energy Efficiency: Improved heat transfer and reaction kinetics can reduce energy consumption.
2. Applications in API Synthesis
1. Reaction Optimization
- Rapid Screening: Flow chemistry allows rapid screening of reaction conditions to identify optimal parameters.
- Parameter Control: Fine-tuning of reaction parameters in real-time to achieve desired outcomes.
2. Complex Multi-Step Synthesis
- Sequential Reactions: Integrating multiple reaction steps in a single flow system to streamline complex API syntheses.
- In-Line Purification: Combining synthesis and purification steps within the flow system to enhance efficiency.
3. Handling Unstable Intermediates
- On-Demand Generation: Producing reactive intermediates on-demand and consuming them immediately to avoid stability issues.
- Minimized Degradation: Reduced exposure time for unstable intermediates, leading to higher yields and purity.
4. Stereoselective Synthesis
- Enhanced Selectivity: Precise control over reaction conditions can improve stereoselectivity, important for chiral APIs.
- Continuous Monitoring: Real-time monitoring of reaction progress to ensure consistent stereoselective outcomes.
3. Technologies and Equipment
1. Microreactors
- Design: Microreactors with microchannels provide high surface area-to-volume ratios, enhancing heat and mass transfer.
- Materials: Made from materials like glass, stainless steel, or polymers, suitable for different types of reactions.
2. Continuous Stirred Tank Reactors (CSTRs)
- Operation: Continuously fed with reactants and allow for well-mixed reactions, suitable for longer residence times.
3. Tubular Reactors
- Flow Characteristics: Long, coiled tubes that maintain plug flow characteristics, providing uniform residence times.
4. Integrated Systems
- Automation: Automated systems with integrated pumps, reactors, sensors, and controllers for real-time adjustment and optimization.
- In-Line Analysis: Incorporation of analytical tools such as NIR, UV-Vis spectroscopy, and HPLC for real-time monitoring and quality control.
4. Challenges and Considerations
1. Technical Challenges
- Clogging: Managing clogging issues, particularly with solid-forming reactions.
- Pressure Drop: Controlling pressure drop across the reactor, especially in high-viscosity reactions.
2. Process Development
- Transfer from Batch to Flow: Developing methods to translate batch processes to continuous flow while maintaining or improving yield and purity.
- Optimization: Continuous optimization of flow parameters to ensure consistent production quality.
3. Regulatory Compliance
- Quality Assurance: Ensuring that flow chemistry processes meet stringent regulatory requirements for API manufacturing.
- Documentation: Comprehensive documentation and validation of continuous flow processes for regulatory approval.
5. Case Studies and Industrial Examples
1. Case Study 1: Improved Yield and Purity
- API Synthesis: Implementation of a flow chemistry process led to a significant increase in yield and purity for a specific API, compared to traditional batch synthesis.
2. Case Study 2: Enhanced Safety
- Hazardous Reaction: A hazardous nitration reaction was safely scaled up using continuous flow, with improved control over exothermic conditions.
3. Industrial Implementation
- Pharmaceutical Companies: Adoption of flow chemistry by major pharmaceutical companies for the commercial production of APIs, highlighting its scalability and efficiency.
Conclusion
Flow chemistry represents a transformative approach in API synthesis, offering numerous advantages over traditional batch processing, including enhanced control, improved safety, and increased efficiency. By leveraging advanced technologies and continuous processing, flow chemistry is poised to play a crucial role in the future of pharmaceutical manufacturing, driving innovation and sustainability in API production.